GEOTHERMAL
For over 30 years, the Geothermal Group at EGI has been engaged in conducting basic and applied geoscientific research for the geothermal, petroleum, and mining industries, government agencies, and international organizations.
EGI’s geothermal group can also assist companies in developing and managing both high and low temperature resources through R&D to uncover the causes of particular industry problems. Browse our capabilities, resources, and expertise for details and ways to get involved.
Using cutting edge tools, our staff have deep expertise in applied geoscience and engineering required to explore, drill and develop geothermal resources. It is now on the forefront of Enhanced Geothermal Systems (EGS) technology with the Utah FORGE project, which aims to produce power from hot dry rock.
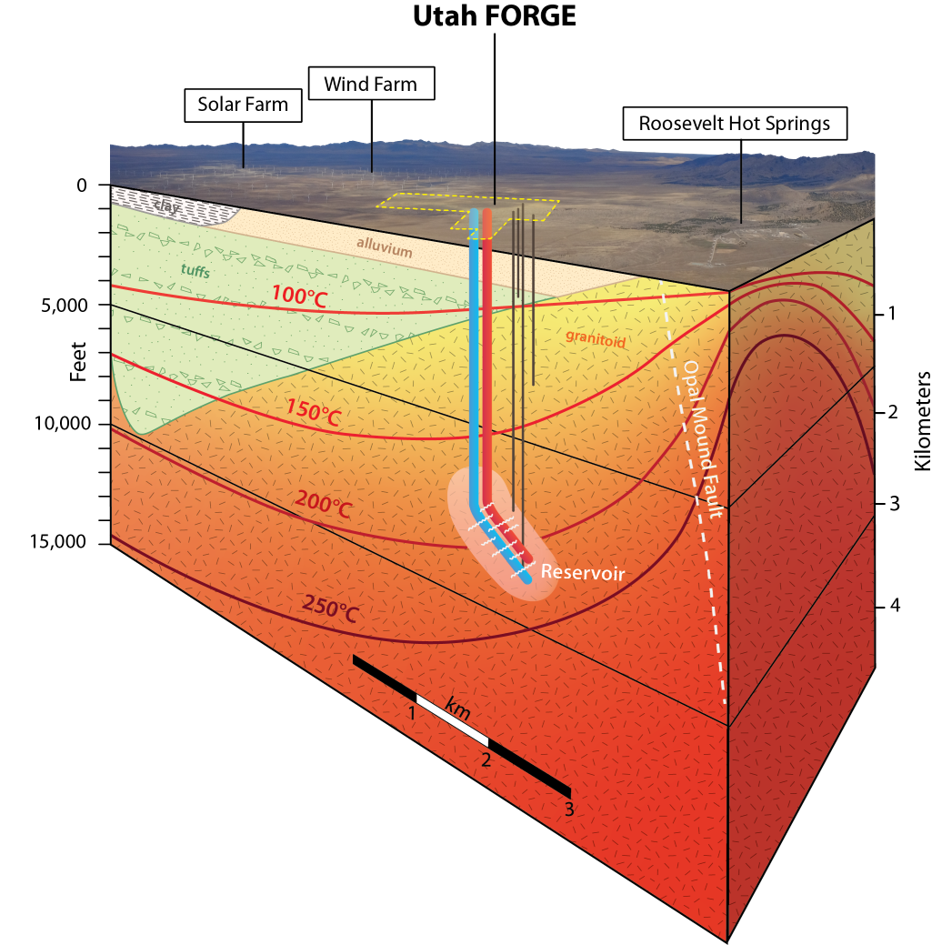
Geologic investigations span a broad range of topics and scales. Geologic mapping, lithologic studies of drill core and cuttings, evaluation of mineral distributions, interpretation of satellite images, fluid-inclusion investigations and the structural analysis of faults and fractures are among the various studies frequently incorporated into geothermal exploration and development programs.
Taken together, the results of these investigations provide the basis for the conceptual models used to site production and injection wells. These models are continually updated and refined as the initial geothermal targets progress from the prospect stage to a fully developed field. At EGI, the heart of its geologic investigations is the Geothermal Sample Library, which houses drill samples from geothermal systems throughout the world.
BROWSE OUR CAPABILITIES, RESOURCES, AND EXPERTISE FOR WAYS TO GET INVOLVED
EXPERTISE
-
- More than 30 years experience in the geologic, geophysical, and geochemical assessment of geothermal and mineral resources throughout the world.
- Development of high-temperature chemical tracers for geothermal and groundwater systems. Our facility houses a fully equipped laboratory for the development of geothermal and groundwater tracers. Most of the chemical tracers currently in use by the geothermal industry have been developed by EGI.
- Collection and interpretation of electrical, magnetic, and gravity data and the development of software for electrical geophysical methods.
- Determination and interpretation of hydrothermal alteration and evaluation of the thermal evolution of geothermal reservoirs. Well equipped petrographic, X-ray diffraction, and fluid inclusion laboratories are available on the premises.
- Evaluation of subsurface permeability in fracture controlled reservoirs and geologic mapping in granitic, volcanic, and sedimentary terrains.
- EGI’s Geothermal Sample Library of core from across the globe is the world’s largest, and constitutes a tremendous and irreplaceable facility for research and instruction.
GEOLOGIC STUDIES
Geologic investigations span a broad range of topics and scales. Geologic mapping, lithologic studies of drill core and cuttings, evaluation of mineral distributions, interpretation of satellite images, fluid-inclusion investigations and the structural analysis of faults and fractures are among the various studies frequently incorporated into geothermal exploration and development programs.
Taken together, the results of these investigations provide the basis for the conceptual models used to site production and injection wells. These models are continually updated and refined as the initial geothermal targets progress from the prospect stage to a fully developed field. At EGI, the heart of its geologic investigations is the Geothermal Sample Library, which houses drill samples from geothermal systems throughout the world.
CHEMICAL TRACER DEVELOPMENT
Tracking the flow of fluids injected into petroleum, groundwater, and geothermal reservoirs can be achieved with tracers. They must be nontoxic, very detectable, available and affordable in bulk, naturally absent from the reservoir and, in the case of geothermal reservoirs and some petroleum reservoirs, very thermally stable. Tracers meeting all these criteria are currently being developed at EGI. Following years of research funded by the Department of Energy, EGI developed and demonstrated both in the laboratory and in the field a family of fluorescent compounds, the naphthalene sulfonates, that have since gained widespread application by geothermal operators around the world. Many of the properties that qualify these chemicals as excellent geothermal tracers have also been used to explain their successful application to tracer tests in petroleum water floods and steam floods. In addition to the naphthalene sulfonates, which partition exclusively to the aqueous phase, EGI has developed and demonstrated techniques for the use and analysis of families.
Primary Equipment at EGI’s Tracer Lab
- 2 high performance liquid chromatographs (HPLC) with fluorescence, absorbance, & conductivity detection
- 1 ultra performance liquid chromatograph (UPLC) with fluorescence & absorbance detection
- 2 gas chromatographs (shared with the petroleum lab) with SPME and a Perkin Elmer ATD650 thermal desorber; detectors include mass spec, FID & ECD
- 3 high pressure/temperature flow reactors for simulating flow processes and for measuring rate kinetics (e.g. tracer sorption & mineral dissolution) in wellbores & in fractured geothermal reservoirs
- 3 high pressure/temperature (400° C/5000 psi) batch reactors
- 1 dynamic diverter reactor (DDR) for testing novel fluid diversion agents for use in engineered geothermal systems (EGS)
- Electronics laboratory for the design, fabrication and testing of high temperature geothermal well bore tools
FLUID INCLUSION ANALYSIS
Hydrocarbon applications for fluid inclusions and mineral investigations provide information for:
- diagenesis and cementation
- timing of oil migration (pre or post cement)
- maturity of the oil number and kind of mobile phases
- temperature of entrapment salinity (source) of the aqueous phase
- composition of the volatile phase
- burial history
Major and minor gaseous species are identified from the inclusions by crushing or thermal decrepitation; noble gases are released by crushing and measured/identified. Samples are analyzed by mass spectrometry and document the presence of magmatic, crustal, and meteoric components in the trapped fluids. EGI’s fluid inclusion lab has a Linkam THSMG 600 heating and freezing stage mounted on a Nikon Optiphot microscope. The stage is equipped with 40 and 80x long working distance lenses. The inclusions can be viewed under plain or fluorescent light when the identification of oil-bearing inclusions is required.
INSTRUMENTATION
Although familiar with numerous electromagnetic exploration and monitoring techniques, we have concentrated on the magnetotelluric (MT) method due to its superior depth of exploration and the relatively advanced state of understanding in its numerical simulation. The physics of this method also forms the basis for new-generation array data collection modes arising in the contracting industry.
The MT method uses naturally occurring EM wave fields as sources to probe the electrical resistivity structure of the subsurface. The fields are at high frequencies (short periods, T < ~1 s) due to regional and global thunderstorm activity. At lower frequencies (long periods), the fields result from regional electric current systems in the ionosphere and magnetosphere originating from fluctuations in solar wind intensity.
Due to the remote nature of the sources and the high index of refraction of the Earth relative to the air, these EM fields usually are assumed to be planar in geometry and to propagate vertically downward. However, the scattering of these waves by subsurface structure may be arbitrary in polarization, requiring a tensor measurement of the fields for full representation. The propagation of EM waves on the Earth is diffusive, so high-frequency signal penetrate a relatively shallow distance while low frequencies penetrate farther. The collected EM time series are decomposed to spectra, ratioed, and finally reduced to the fundamental response of interest – the tensor MT impedance Z. Simple expressions of apparent resistivity (ra), smoothed version of Earth resistivity below site) and impedance phase (j) are typically used for presentation. This scattering response is measured at numerous sites over the surface, often in dense profiles, and over a broad frequency range (e.g., 1 kHz to 0.001 Hz, and even lower). Numerical simulation algorithms have emerged to reproduce the MT response of specified structure (forward problem), or to generate conservative images of the subsurface directly from the data (inverse problem). Finally, resistivity models are interpreted in terms of structures representing fluid content, temperature, melting, and solid phases such as graphite or sulfides.
Our new MT system design combines simultaneous multi-band collection to help ensure long data records in the problematic MT mid-band, with high-speed, longer distance telemetry to provide real-time remote referencing with signals completely outside the influence of geothermal production noise. Improved capabilities for bandwidth limiting through analog filtering and the use of 24bit A/D converters to increase dynamic range are important new capabilities that will aid the acquisition of MT data in the presence of substantial locally generated noise. Finally, our real-time processing algorithms provide the highly desirable capability to assess data quality as it is acquired to optimize acquisition while on site.
These are based on discrimination by multiple coherence at each frequency and can be defined flexibly among different field subsets and independently for each MT polarization mode. New processing approaches which consider two-source (conceptually multi-source) transfer functions are to be generalized for application to geothermal systems. An electronics shop is maintained at the University of Utah/EGI which is used to fabricate, integrate, and test MT and other geophysical equipment. The shop contains electronic test equipment and machine shop equipment sufficient to carry out all aspects of construction and testing, except for printed circuit board layout and fabrication prior to component loading, which is farmed out to appropriate vendors. This shop has been operated and maintained continuously for more than fifteen years during the development and operation of our in-house MT instrumentation.
An example response acquired using the UU/EGI MT system appears here to exemplify possible data quality with our platform and illustrate the method’s basic physical principles. This site in central Nevada was not taken in an active geothermal system but reflects mainly regional stratigraphic and Basin-Range structural elements. Two panels of data points are plotted: the upper being apparent resistivity (ra) derived from two of the tensor impedance elements, and the lower being phase of the elements (j). The latter tends to be proportional to the slope of the former versus period (T). The two elements approximately represent current flow along geological strike x (NNE) (xy quantities) and across strike (yx quantities).
Data values at individual periods are plotted as 1 s.d. intervals which generally are quite small. Estimate quality was improved significantly by sorting EM field spectra at each period according to multiple coherence and using a simultaneously recording site several kilometers away as a remote reference for noise cancellation. The sounding plots exemplify the smooth nature of the responses w.r.t. T, in keeping with the diffusive nature of EM propagation in the Earth. This occurs despite the probability that some structures in the Earth have sharp boundaries, and is at the heart of the ill-posed nature of the MT inverse problem. Note the divergence of the ra curves as period increases, which is due to the increasing influence of conductive valley sediments lateral to the site. The mid-period minimum reflects influence of conductive, mid-Paleozoic shales while the decrease in ra at the long periods is caused by thermally-induced conductivity increases in the lower crust.
FIELD CAMPAIGNS
Dixie Valley, NV, contains a classic Basin-Range geothermal system that is currently producing and has been the subject of substantial research. Under new funding from DOE/OGWT, we will be undertaking detailed MT surveying of the system for two main purposes.
First, we wish to assess whether lateral permeability variations known to exist in the system can be mapped with useful accuracy according to electrical resistivity expression. Second, current power production at Dixie Valley generates strong EM fields which interfere with the low-level natural signals we attempt to measure in the MT method. MT signals in fact were overwhelmed for sites close to the power plant and we will attempt to overcome this using multi-transfer function methods we are developing. An initial transect of sites has been acquired across Dixie Valley and the Stillwater Range for background control in the upcoming, contiguous bipole profiling.
One of our most notable successes with the MT method was in our transect across the South Island of New Zealand, an active compressional orogen. Forty-one tensor soundings, twelve of which required helicopter support in the Southern Alps, were collected under support from the U.S. National Science Foundation using the University of Utah/EGI MT system. Two inversions of the cross-strike (transverse magnetic or TM mode) data, one using our a-priori approach and the other using the damped curvature approach of Rodi and Mackie, each show a pronounced, concave-upward conductor originating in the lower crust and shallowing towards the principal forethrust (Alpine Fault) and backthrust (Forest Creek Fault) zones of the S. Alps.
Inversions including the along-strike (transverse electric or TE) data (not shown) imply strong conductivity anisotropy with the more conductive direction along strike. The conductor is interpreted to represent fluids formed by prograde metamorphism in the thickening crustal root zone, and their expulsion towards the surface in uplifting, deep crustal schist. Near-surface approaches of the conductor correspond to outcrops of Late Cenozoic-present, mesothermal gold deposits, so that MT is glimpsing the creation and transport of this globally-significant class of ore fluids in nearly real time.
NUMERICAL SIMULATIONS
Numerical simulation of Earth EM responses using computers is of great importance in effective survey design and translating observed data to reliable estimates of subsurface structure. Survey design and fundamental response understanding is achieved through the process of forward modeling, where the response at the receivers due to a user-specified resistivity structure is predicted numerically. Forward modeling techniques are complicated by the very non-linear relation between the response and the physical property (resistivity), and by the discontinuous nature of the electric field across resistivity contacts. Direct structural estimates from the data are accomplished through inversion, which typically must be iterative due also to the non-linear character of the physics. The diffusive nature of EM geophysics requires the application of solution constraints, to avoid model artifacts not required by the data.
Inversion of geoelectrical profile data at EGI makes use of finite element forward modules developed at Utah over many years. The forward modules have been used in many publications where principle features of data sets have been reproduced in trial-and-error fitting. Current directions in two-dimensional (2-D) inversion emphasize automatic, iterative fitting of observations while constraining the solution to remain as close as possible to a-priori structural constraints (after Tarantola). For the 2-D problem, it is practical to generate parameter step estimates in the non-linear iterative procedure using the Gauss-Newton step.
Our main area of research is in the definition of solution constraints and their implementation within the parameter covariance matrix of the non-linear step equation. Instead of using the popular but brute-force damping of solution slope or curvature, we instead exploit natural parameter correlations in diffusive EM such as conductivity-layer thickness to produce smooth, conservative solutions. Computer resource demands and solution conditioning are improved by allowing resistivity parameters (pixels) to grow both laterally and with depth in the Earth, in order to equalize their influence on the surface response through the concept of EM scaling.
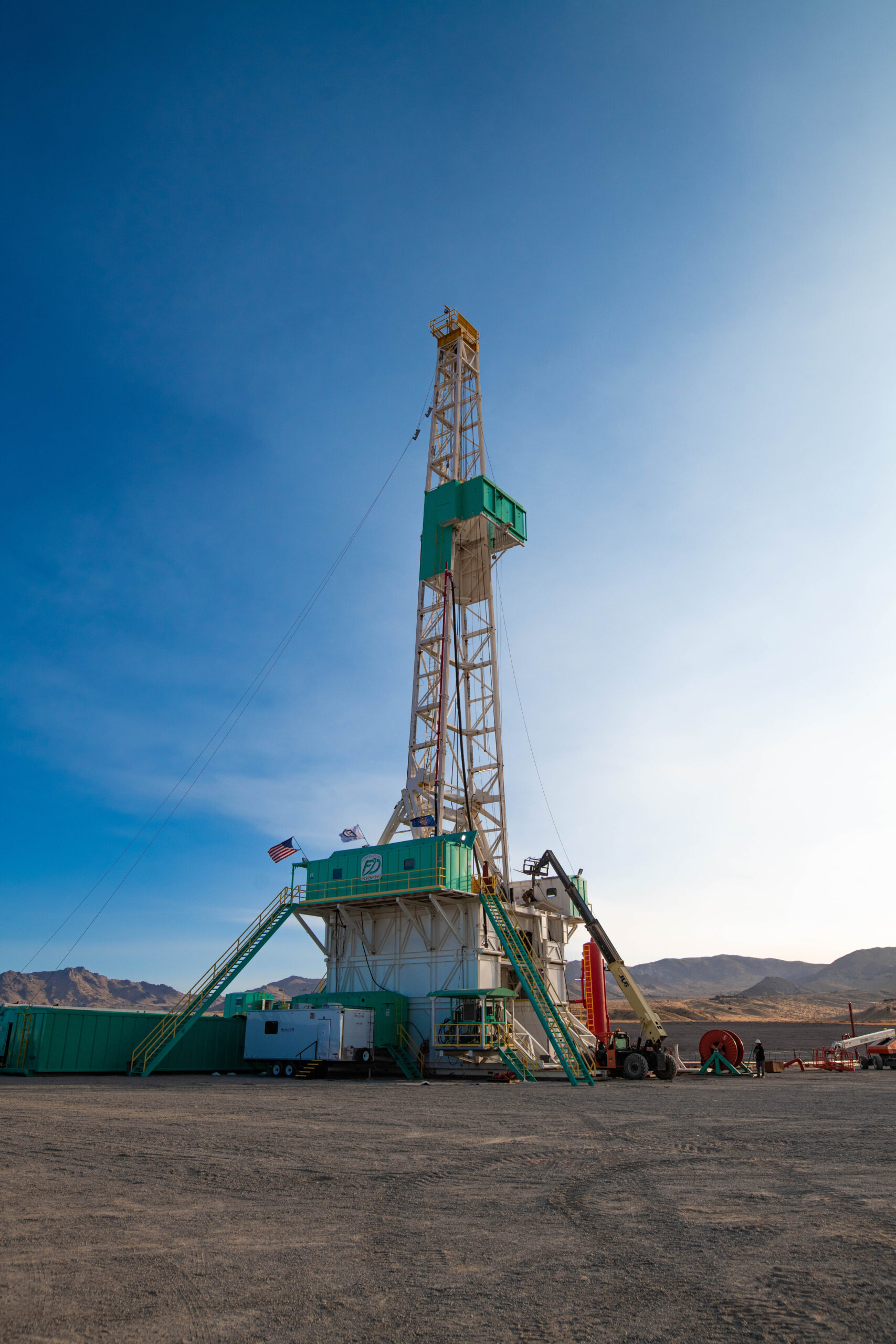
Utah FORGE underground field laboratory
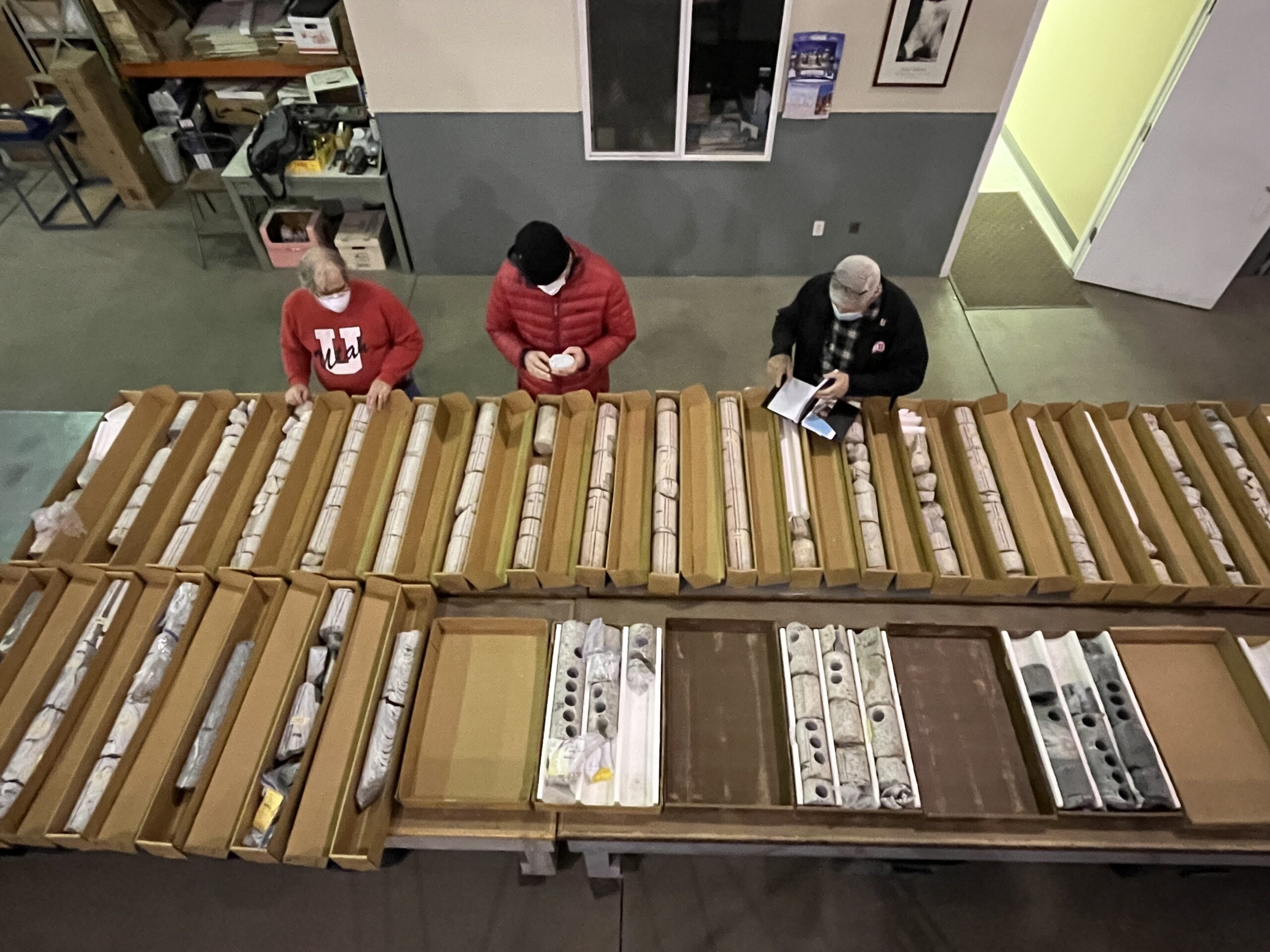
Core samples taken from Utah FORGE site.
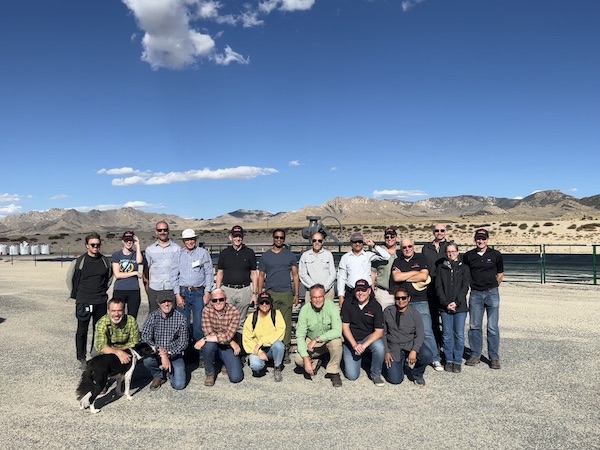
Tour of Utah FORGE site during the 2022 Corporate Associates Technical Conference.
CORPORATE ASSOCIATE MEMBERS
EGI offers Corporate Associate members a competitive advantage in a highly competitive industry with our diverse staff of expert geoscientists, engineering researchers and innovative technical staff.
EGI’s Corporate Associate members gain access to over $850 million of regional and thematic research for only a fraction of their investment.
Expert Geoscientists
Engineering researchers
Innovative technical staff
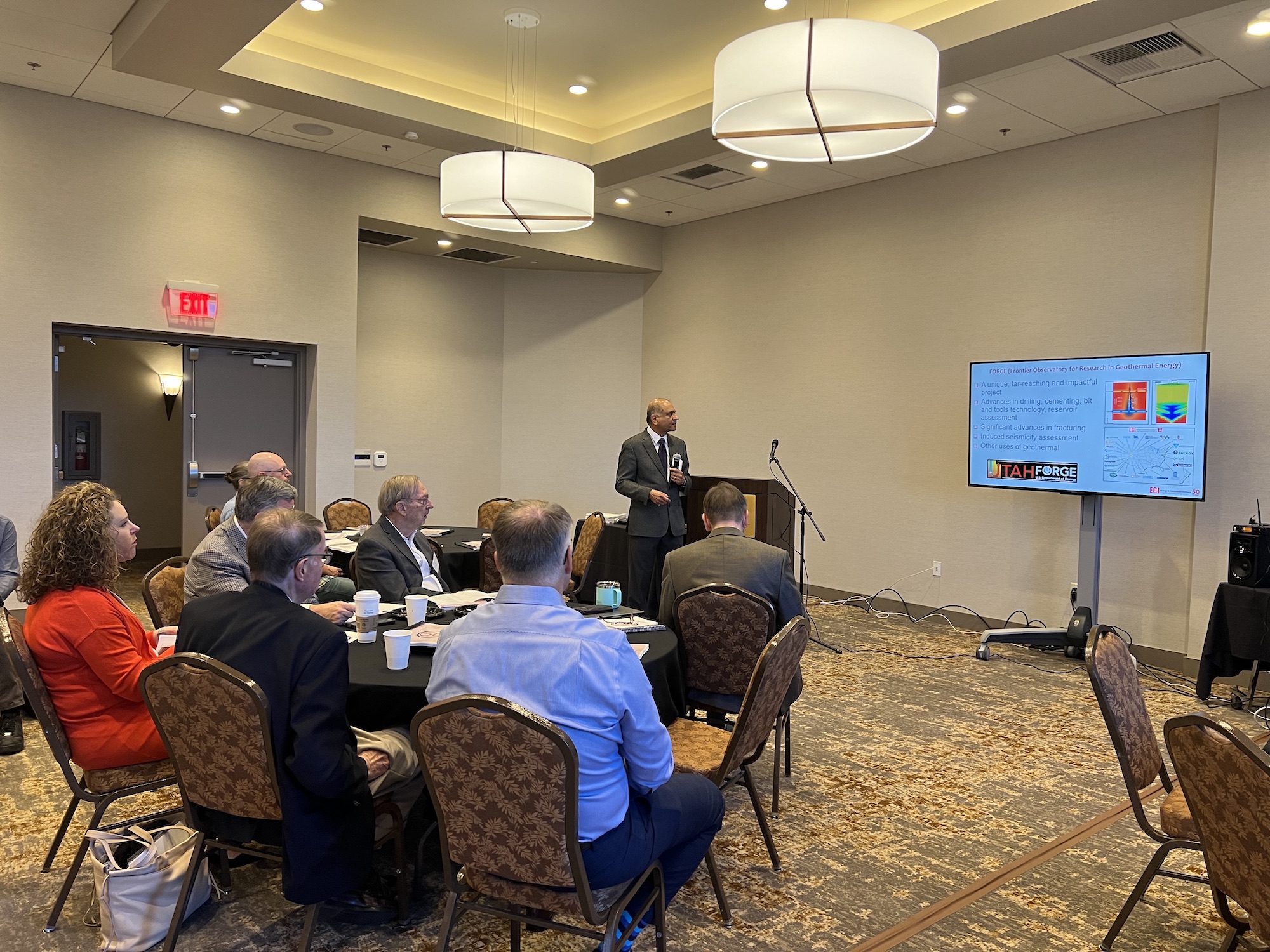
CONTACT US ABOUT BEING A CORPORATE ASSOCIATE MEMBER
ContactEGI@egi.utah.edu | (801) 581 – 5126